- Joined
- Mar 26, 2018
- Messages
- 2,725
I did quite a bit of reading om practical machinist on fixing the DC mechanism in these. Two possible routes became obvious, basically replacing the DC controller, or replacing the DC motor with an induction (standard AC) motor and vfd.
The monarch 10EE is a bit unusual for a lathe in that the spindle is ungeared. It is a straight through shaft and however fast the belt drive turns that end is how fast the chuck or whatever is mounted on the working end will turn. And yet you get this incredible range of useable speed, like 10 rpm to 4000 rpm on mine. This is done via a DC motor which has torque over a fairly broad range of rpm, plus a reduction gear mounted on the motor, giving direct motor rpm out, or IIRC a 5:1 reduction.
The challenge with the induction motor plus vfd route is even with a good vfd, 10-4000 rpm on the spindle is a 400:1 range of rpm to operate. Many vfd machines we see today run 20-2000 rpm, 100:1, by using two or three geared speed ranges. Also, a vfd driven motor roughly creates the same torque at speeds less than the design speed of the motor. HP = rpm * torque * constant, where constant is a number which depends on units used. So if torque is constant, than as rpm drops, so does HP. On a lathe if you are looking for a certain SFPM cutting speed, you go slower on bigger (larger diameter) parts. Larger diameter means less leverage for the motor, so the motor needs more torque to give the same force at the cutting tool at that SFPM. So a VFD isn’t to optimum configuration for getting power to a machining tool as we reduce rpm below the motors rated speed. On the other end, as we increase rpm above the motor’s rated speed, vfd’s have to reduce the torque as speed increases (above the 60Hz speed of the motor), to keep the motor from exceeding its rated HP output. This matches well with what we want for most machining. Turning faster is associated with smaller endmills or smaller diameter workpieces in the lathe.
One takeaway from this is if you have a vfd machine with multiple speed ranges and you need the most cutting power, you should opt for the lowest speed range that will give you the desired cutting speed. So if your lathe has ranges 50-500 and 200 to 2000, and 400 rpm is your desired cutting speed, you are better off using the 50-500 rpm range. The high range will of course still give you the option of 400 rpm, but less cutting power. Just like you can use third gear at 20mph in your manual transmission car, but if you want to accelerat, downshift to 2nd or first.
Ok, rhe point of all that explanation is that a VFD driven motor isn’t going to give you good cutting performance over a spindle rpm range of 10-4000 rpm. There are two ways to help this along. One is to use some gearing. The other is to use an oversize motor.
The 10EE does have that reduction gear in its design. The challenge is that the reduction gear is part of the DC motor assembly, between the DC motor and the drive pulley. So you can’t just take the pulley off and put it onto a VFD driven motor without losing the reduction gear. Quite a few 10EEs out there have been converted in just that way, the reduction gear is discarded along with the old motor. Even with a ridiculously oversized motor (commonly up to 10HP) the low rpm performance suffers. Singke point threading is one area that this can become an issue. Since that is one area where the classic 10EE shines, it is a shame to sacrifice capabilities in that area.
A very good solution to this is to re-use the original reduction gear unit. This is somewhat complicated to do in that the original DC motor is not a simple keyed shaft but rather a splined shaft into the reduction gear. This has been solved many ways, such as making a keyed shaft to spline adapter, or more commonly just cutting off the spline end of the original DC motor and putting a keyway on the cut end, along with a coupling.
So, a large (5-10HP) AC induction motor, reduction gear adapter and VFD is probably the cleanest solution. That’s not necessarily cheap, unless you can find a bargain on a good used AC motor and suitable VFD.
The option I took was to preserve the whole motor assembly and build a new DC motor control system. In retrospect that certainly wasn’t the quickest solution to a functional lathe, and is only practical in that I do have a background that includes electronics. I also wanted to run the whole thing off single phase rather than a phase converter.
One thing to think about is modern high end VFDs will offer Field Oriented Control (FOC). When you use this control mode with an encoder on the motor, you can see 1000:1 torque range.
Here is an excerpt from an Allen Bradley whitepaper (publication DRIVES-WP002A-EN-P).
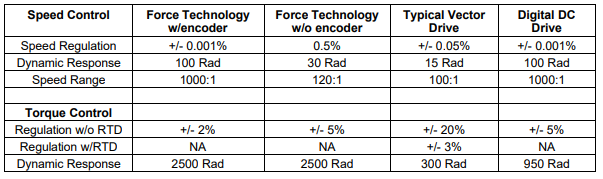
Note that Field Oriented Control (FOC) is branded by AB as "Force Technology". Without an encoder, you get about a 20% gain on a sensorless vector controlled (SVC) VFD. Adding the encoder gets you to the 1000:1 constant torque speed range, in line with a DC drive, as well as much better speed regulation. They also offer better torque bandwidth and regulation than a comparable DC drive.